- Posts
- Storage
Thermal energy storage
Hugues Defreville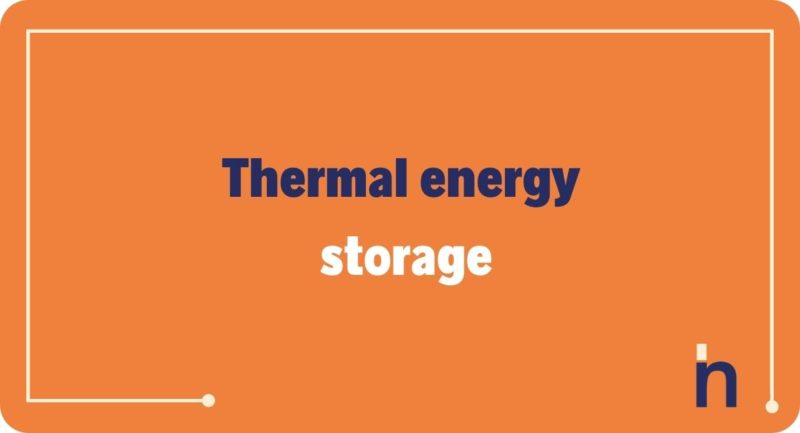
Energy storage: challenges and solutions
As we presented in our recent article on renewable heat, mankind’s energy needs are divided between electricity, transport, but also and mainly heat, or thermal energy.
The issue of storage concerns all these energy uses, heat as well as electricity: in short, it consists of “accumulating” energy for later use. However, the production of renewable energy faces a major problem: the variability and intermittency of the renewable resource used. This is particularly true of solar energy, which has multiple variability, both daily (weather) and seasonal.
Knowing how to store energy is therefore a key point in the energy transition, an essential issue for the massive introduction of renewable energy sources (RE) into our energy mix. And this is true for both thermal and electrical energy!
Electricity: the most familiar yet most complex storage solutions!
Today, the public is most familiar with the solutions dedicated to the storage of electricity.
However, while electricity is a very practical and efficient vector for transporting energy, its accumulation remains particularly difficult, and generally requires transformation to be stored in another form. The most common forms of electricity storage are those based on electrochemical solutions, using batteries (lead-acid, sodium-sulphur, lithium-ion, etc.). We use them on a daily basis, particularly for mobile applications (telephones or laptops).
Historically focused on low power applications, systems with higher capacities have been developing for a few years now, particularly for mobility applications (electric cars, with pioneering manufacturers such as Tesla and Nissan, followed today by all the manufacturers on the planet), but also with the development of even larger systems, making it possible to take part in the balancing of supply and demand when there is a surplus of renewable electricity production.
For example, the Victorian Big Battery, the largest battery in the world, installed in Australia and financed by the French independent producer Neoen, is a good example. [1] from 350 to 400 MW of power, based on the “Megapack” solution of the American company Tesla).
However, these solutions mainly meet storage needs of relatively short duration (a few hours to a few days for small powers) and can therefore only cover relatively small or medium quantities of energy. Finally, their cost remains relatively high and their lifespan is limited by the chemical degradation of the reactions. Although this battery sector is in full expansion, there are also other electricity storage sectors that allow for greater accumulation capacities, but their development potential currently appears to be low or limited:
- Mechanical gravity solutions, such as pumped storage stations (PETS): this is the main solution used worldwide for massive energy storage in power plants. However, its development potential is currently limited by the number of sites suitable for its installation (in France in particular)
- Compressed air energy storage (CAES) can also be used to store large amounts of power and energy over several weeks. However, its development potential is also limited by the sites required for its installation
- Flywheel technology is also mature, but only allows a very short storage time and a limited amount of stored energy.
Yet the electricity storage option that is currently attracting the most interest, alongside batteries, is the use of hydrogen (and to a lesser extent other “power to gas” processes). This consists of producing hydrogen by electrolysis of water, using renewable electricity (photovoltaic solar panels, wind turbines, etc.). It is then possible to store this hydrogen, inject it into the gas transport network, power vehicles or even convert it back into electricity via a fuel cell.
The government and industry currently seem to have identified hydrogen as the most promising method of passive inter-seasonal storage, even if its cost is still very high and its large-scale development is not expected before 2035.[2]
Did you know? Since 2020, NASA has launched the “Watts on the Moon” competition to the general public (in the USA) to find new options for distributing, managing and storing the solar photovoltaic (electrical) energy that would be produced on the Moon! With nights lasting 350 hours in a row, extreme temperature changes… storing electricity on the moon is a real challenge, even for NASA!
Heat: simple and robust solutions, allowing for the massive development of renewable and recovered production
Focusing on the issues of thermal storage, one should note that, in addition to the variability and intermittency of certain renewable resources such as solar energy, large heat consumers can also see their thermal needs vary greatly, whether for foreseeable reasons (variable production load of an industrial site or start-up of the heating of a district network), or unforeseeable technical problems (machine breakdown, climatic hazard, etc). climate change, etc.).
Moreover, each major heat consumer is supplied by a dedicated and independent production and distribution system (and not via a local, national or even international network as is the case for electricity). Here, the challenges are even more obvious, and must necessarily be implemented for each consumer, in order to match variable and intermittent production with consumption that is no different!
As you will find out in the rest of this article, there already are “massive” thermal energy storage solutions! They are simple and robust, they use mastered technologies and are particularly efficient economically and environmentally. They can be used to store energy over periods of several days (known as “daily” storage) or over several months (known as “inter-seasonal” storage).
At Newheat, we almost systematically integrate a thermal storage solution into our projects. These systems are the cornerstones of our power plants, allowing us to provide our customers with energy that meets their needs and can adapt to technical and climatic contingencies.
Types of thermal storage systems: sensible, latent, thermochemical
There are 3 methods, based on different physical phenomena:
- “Sensitive” storage: This method is the simplest and most widespread. It consists of heating a liquid or solid material, without it changing phase (melting, vaporisation, etc.). The material to be used is chosen according to the temperature level required by the application:-below 100°C, liquid water is the most widely used compound. It is non-toxic, inexpensive and (for the volumes involved) very abundant. Solids, such as rock or concrete, can also be used.-Above 100°C, liquids such as molten salts or organic oils can be used, which can reach up to about 350°C for oils and 800°C for molten salts. However, their cost and environmental risks are major issues limiting their use.Systems using solids such as high-temperature concrete or refractory ceramic (up to 1000°C) can also be used. The volumes required are then greater than for liquid media.
- “Latent” storage: aims to exploit the latent heat released (or absorbed) during the phase change of a material. The material used will change from a solid to a liquid state, thus absorbing a large amount of thermal energy. It will then be able to release this heat in the reverse transition phase, when it solidifies. The energy required to change the phase of a material from solid to liquid is called the “enthalpy of fusion”. To achieve this, specific materials (with the highest melting enthalpy) called “Phase Change Materials” (PCMs) are used. The main PCMs used in large systems are paraffins, fatty acids and hydrated salts. Although their energy density is higher than that of so-called “sensitive” systems, they are often limited in terms of charging and discharging power. They are still not very developed
- “Thermochemical”: it uses the reversibility of a chemical reaction which is, depending on the direction of the reaction considered, either endothermic (heat consuming) or exothermic (heat emitting). A distinction can be made between:
-Storage by Adsorption/Desorption involving a solid/gas couple. Adsorption corresponds to the fixation of gas molecules on the surface of the solid. This phenomenon is exothermic and therefore releases heat. Desorption corresponds to the release of gas molecules from the surface of the solid. This phenomenon is endothermic, and therefore consumes heat (energy storage). The main adsorbent materials used are silica gels, aluminophosphates, silicophosphates and zeolites.
-Storage involves a chemical reaction between different salts and water (hydrates). The salt, initially in a solid state, decomposes/dissolves under the effect of heat. This is the energy charging stage. When the components of the salt are brought together again, it is reformed into a solid state. This process is exothermic and therefore gives back the heat supplied, this is the discharge stage.
Such systems, however, require complex technologies and are not yet widely developed.
At Newheat, although we are open to all technologies and follow scientific advances with great interest, we have chosen to focus initially on sensitive storage systems for applications with temperatures below 100°C, whose maturity and robustness is no longer in question.
In the remainder of this article, we will focus on 3 options that seem to us to be the most technically and economically efficient, environmentally friendly and have many references: these are the solutions that we are already deploying in our projects!
Thermal energy “daily” storage (applications below 100°C): Stratified storage tanks
Storing heat in the form of hot water in large tanks, like a giant “thermos”, is particularly efficient technically and economically.
These large tanks can be made of concrete or steel (the latter allowing the most competitive installations) and have a unit volume of up to 10,000 m3 (or more). They are then covered with an insulating material such as rock wool or glass wool and are finally protected from bad weather by metal cladding.
To give an order of magnitude, a 3,000 m3 (or 3 million litre) tank has the following dimensions:
- Height of 12 metres (equivalent to a 4-storey building)
- Diameter of 20 metres
- Volume equivalent to an Olympic swimming pool
This is a dimensioning that we use in the implementation of our large solar thermal plants at Newheat! Like the one at the Malteries Franco-Suisses.
photo credit : Axéréal
A tank of this size can store about 150 MWh of thermal energy (assuming a “useful” storage temperature range of 40°C), which corresponds to several days of summer production from a power plant with a peak power of 10 MW. This is why we call this type of storage “daily”. It can in fact make it possible to compensate for the drop in solar resources over a few consecutive days, but will be insufficient to cover the deficit in sunshine during the winter period.
Note that these systems use water in a “closed loop” (initially cold, it is heated by the renewable heating, then cooled when it is delivered to the consumer, and everything starts again in a potentially infinite cycle). Once the system is filled, there is no “consumption” of water, and it can be reused without pollution at the end of the installation’s life.
Controlling stratification, a central issue in storage system performance
In terms of energy efficiency, the levels of heat loss from this type of tank are very limited (a few % maximum of heat loss), even for periods of several weeks, provided, however, that the movement of water within the tank is controlled in order to preserve its good thermal stratification.
How do can we define “thermal stratification”? The phenomenon of thermal stratification corresponds to the “ordering” of the temperatures of a fluid within a container according to their density. The warmest temperatures are found at the top of the vessel (the “hot” fluid being less dense) and the coldest at the bottom of the vessel (the “cold” fluid being denser).
In case of bad stratification, for example with the appearance of convection phenomena or “mixing” of the water (created by a flow of water entering or leaving the tank), the temperatures mix and it becomes uniformly “tepid”. This water is then much less interesting, and will no longer be able to supply a consumer who only requires it to be ” hot ” at a high temperature level.
To measure this performance, the notion of “exergy efficiency” is used, which measures the “quality” of the stored thermal energy, i.e. the part that can be used for a given purpose, above a certain temperature.
To ensure good stratification and to maintain the finest possible thermocline (transition zone delimiting a hot water zone floating above a cold water zone) and to avoid this mixing and exergy “destruction”, it is important to :
- Design the system so that the supply (from the solar power plant) and use (by the consumer) of hot water is from the top of the tank, and the withdrawal (to the solar power plant) and return (after supply to the consumer) of cold water is from the bottom of the tank.
- Plan and design hydraulic systems to control hydrodynamic phenomena within the tank (e.g. by “breaking” the speed of water entry) to avoid “mixing” all temperature layers. They can be simple deflectors (metal plates directing the flows) or equipment such as diffusion rakes or stratification columns.
Inter-seasonal thermal energy storage (applications below 100°C): Borehole thermal energy storage and Pit Thermal Energy Storage – PTES
While it is always possible to multiply the number of tanks by several thousand m3 to increase the thermal storage capacity, two other solutions that make it possible to conserve heat below 100°C seem to us to be more relevant technically and economically when larger volumes of energy are stored.
In particular, if we are looking to carry out “inter-seasonal” storage, i.e. to accumulate sufficient thermal energy in the summer period to be able to supply heat consumption in the winter period.
Borehole thermal energy storage – BTES
These consist of storing heat in the ground through a series of geothermal boreholes.
This is done using so-called “shallow” boreholes (50m to 300m), the objective of which is not to seek the heat contained in the earth’s mantle or in magma bodies (“deep” geothermal energy can be found at depths of up to 5,000 metres), but rather to seek out soil at a stable temperature of around 15°C.
Once the boreholes have been drilled, the ground can then be ‘charged’ with energy by sending hot water through these boreholes into a system of boreholes(again in a closed loop, with the water returning through a return pipe once its heat has been transferred to the ground). Once the ground is warm, this heat can then be recovered by sending cold water through the boreholes, which then returns warmed by the passage through the warm ground. To work, the soil must be compatible. For example, the presence of rock that is too complex to drill or the presence of water flowing underground that would allow the heat to “escape” are major issues that can limit the interest of these systems.
These solutions are still relevant for a very large part of the territory. In terms of thermal performance, this type of system is particularly efficient up to about 40°C – 50°C. Moreover, the load/discharge power is limited by the exchange surface between the ground and the probes, which is much more restrictive than systems in the form of hot water only, for which it is sufficient to adjust the flow rates.
In the end, according to our analysis, their use is particularly relevant up to 50°C, and for storage capacities in “equivalent water volume” of 10,000 to 100,000 m3 (i.e. 250 to 2,500 MWh for an assumption of a 20°C “useful” storage temperature range). They are regularly coupled with heat pumps to reach higher temperature levels at the cost of additional electricity consumption.
Did you know that in Canada, in the state of Alberta in Ototoks, an entire eco-neighbourhood of 52 homes, the Drake Landing Solar Community, is heated by the sun year-round? In addition to the 800 collectors, an inter-seasonal Borehole Thermal Energy Storage system (as described above) is integrated to store solar heat underground during the summer months and distribute it to each home for heating during the winter months…when the weather averages between -2° and -13°c! This project, unprecedented in the world, meets 95% of the heating needs of each house.
photo credit : DLSC
Pit Thermal Energy Storage – PTES
When water is used as a medium, it becomes interesting, from a certain volume onwards, to move from the construction of tanks with metal or concrete walls to the creation of large “pits” or “pools” dug into the ground.
This type of solution, known as Pit Thermal Energy Storage, consists of making very large excavations, which can cover several hectares and be up to 20 to 30 metres deep.
Once the earthwork has been completed (the excavated soil is used on site, no soil is removed or brought in), a liner is installed on the bottom and sides of the pit, and then a floating insulation cover is added on top. This is the most complex and expensive part of the system and can be up to 1 or 2 metres thick. It consists of different layers of insulation and waterproof membranes and is weighted down with gravel.
The operating principle is then identical to that of a large tank: pipes are installed at different heights within the tank, and once the tank is full, care must be taken to ensure that the temperatures are stratified, with hot water in the upper part of the tank and cold water in the lower part.
In terms of performance, although their heat losses are greater than for insulated tank systems, they remain relatively moderate (20 to 40% losses for several months), and vary according to the temperature levels stored.
As the effects of scale have an important influence on the price of these systems, the use of this type of tank becomes economically relevant from a volume of approximately 100,000 m3. These storage systems are thus relevant for storing from 5 GWh to several tens of GWh (assuming a “useful” storage temperature range of 40°C).
Use of such storage systems, individually or in combination with large-scale solar thermal power plants
There are reliable, mature and efficient solutions for storing heat below 100°C, even for large volumes of several dozen GWh!
Despite their undeniable advantages, they are still very little developed in France and Europe, with the notable exception of Denmark, a country that has been at the forefront of the field for nearly 20 years.
If they are central and necessary elements of all the large renewable heat plants that Newheat develops, these thermal storage solutions can also be used for other types of uses, whether to smooth the load or improve the quality of heat supply service (availability) to a consumer, or to improve the coverage of other variable and intermittent renewable production means (such as MWIPs, household waste incineration units).
At Newheat, mastering them is part of our job, we can help you to implement and finance them, even if your project does not include solar thermal energy!
Would you like to know more? Do not hesitate to contact us!
For the most motivated, do not hesitate to join us to contribute to the IEA (International Energy Agency) working group on large-scale storage systems for heat networks (“IEA – ES / Annex 39”), [3] where Newheatis involved as a coordinator for the work done by contributors from all over the world and shared with the whole profession!
Article references:
[1] https://victorianbigbattery.com.au/
[2] https://www.lemondedelenergie.com/stockage-electricite/2021/01/12/